Fusion Energy Innovation: Accelerated Progress in 2020
The world has a major problem to solve. By 2040, we will need 29 trillion KWh of new energy generation to support 9 billion people on the planet, a whopping 45% increase in electricity demand. Wind, solar and other forms of renewables are well-positioned to play a key role, but their volatile, weather-dependent nature means they are not well-suited to replace other large forms of fossil fuel baseload power. Both short-duration batteries, as well as emerging distributed long duration options, can support limited volumes, but will not be able to offer an economically competitive solution by 2040. In short, to sustain economic growth, while also decoupling from fossil fuels, alternative sources of clean, low-cost, baseload energy will be required.
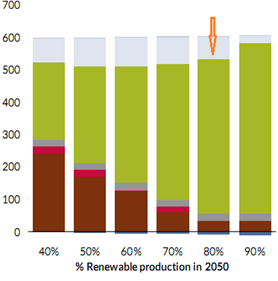
Producing energy from nuclear fusion is widely regarded as the grand engineering challenge of the 21st century. Fusion has the potential to provide an always available, environmentally benign and well-distributed energy solution. It can also provide utility-scale energy on-demand, making it an excellent complement for intermittent renewables and battery storage. Figure 1 highlights how, given any scenario where renewable production s and US-based Commonwealth Fusion Systems serves between 40%-90% of annual demand, nuclear must be part of the solution.
For a very long time now, the narrative for fusion has been that commercialization remains approximately 20-30 years away. This view largely stems from the time, cost and politics involved in producing large, internationally funded projects, including ITER (originally the International Thermonuclear Experimental Reactor) which was conceived back in 1985 and has, to date, cost over $22 billion dollars.
However, the ever-evolving wealth of public data has set the stage for the rise in private-sector activity and has, excitingly, accelerated timelines. Backed by long-term thinkers, impact investors and smart energy industry capital from Equinor, ENI seeking future strategic advantage, a handful of private companies are now aiming to demonstrate operational energy-gain by the mid-2020s and usable reactor designs by early 2030s, with commercial availability thereafter. The graph below highlights the investment activity over the past 10 years, which clearly speaks to the rising interest in the sector. The common line from investors today is that having at least one fusion investment in your portfolio is a necessity.
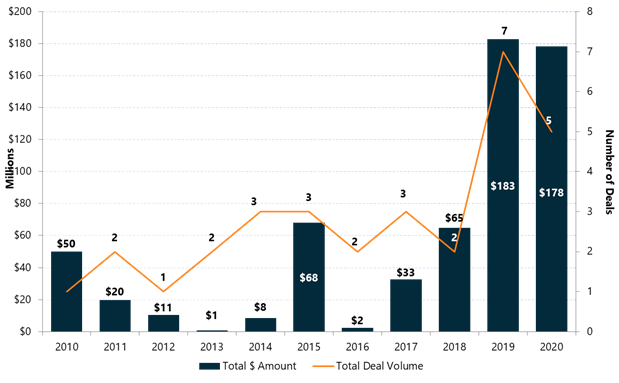
Hearing from key players at our Cleantech Forum Events over the last three years, the tone in the fusion sector seems to be moving from science-based conversations, where questions focus on how, when and if net gain can be achieved (achieving a rate of energy that exceeds the rate of energy injected into the plasma), to that of commercial deployment strategies, as companies look to secure industrial partnerships.
How Enabling Technology Changed the Game
Core science-based challenges have been the key issues for maintaining sustained operation of fusion systems and have prevented sustained net gain. For any of the designs, the ability to effectively sense, monitor and stabilize operation has been limited by the computational power available. However, the quest for nuclear fusion energy is increasingly aided by advancements in machine learning and deep learning artificial intelligence. Modelers can now:
- Successfully forecast disruptions in fusion reactors
- Predict true disruptions within the plasma fuel
- Mitigate any instability effects
- Build in further safety measures
Disruption prediction is critical. The bigger the machine, the bigger the disruption, the bigger the cost. In the $25 billion ITER tokamak for example, any disruptions are expected to be severe, as the project hopes to run initial plasma tests in 2025. Players including Google, and numerous researchers around the world have committed large chunks of public and private funding in order tackle this challenge head on.
Emerging Technology
Although several approaches to controlling and containing a fusion plasma exist, the most developed approaches are magnetic confinement, inertial confinement and magnetized target fusion. The graph below highlights key differences between these technologies, as well as some private and public examples.
Magnetic Confinement Fusion
The most advanced technology, magnetic confinement fusion (MCF), has been the solution of choice for the main internationally led projects including ITER and JET. A large electromagnetic field confines and heats plasma fuel inside a doughnut-shaped reactor called a tokamak. Since early development in the 1960s, over 200 tokamaks have been built. Two private US-based sector innovators, Tokamak Energy and Commonwealth Fusion Systems, have collectedly raised over $350 million in equity funding.
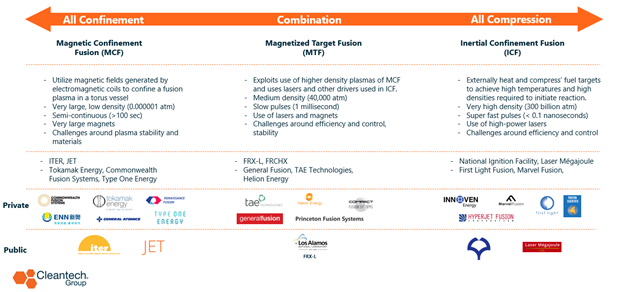
MCF faced backlash due to the high requirements of the superconducting magnet technology. Commonwealth’s innovation is a new, high-temperature superconductor, Rare Earth Barium Copper Oxide (REBCO), which is it using to reduce size and lower cost. The company is collaborating with MIT’s Plasma Science and Fusion Center to build SPARC, a demonstration fusion device to produce net-energy gain, which will ultimately lead to the development of the commercial unit ARC, designed to be connected to the grid in the early 2030s.
Inertial Confinement Fusion
Unlike MCF, inertial confinement fusion (ICF) attempts to externally heat and compress fusion fuel projectiles to achieve very high temperatures at high densities required to initiate the nuclear fusion. For the main ICF reactors in development, high power lasers are used to compress and heat the fuel. UK-based First Light Fusion is the current frontrunner for ICF. Projectile fusion has been considered before but First Light uses a novel target solution to which is low speed and predictable, have developed a novel driver which they claim in 1000x cheaper than other designs. To-date, the company has raised $35 million and is looking to go to market with a business model from manufacturing, licensing, and selling the targets technology to buyers.
Magnetic Target Fusion
Building on the advantages of the other two technologies, magnetic target fusion (MTF) looks to exploit the use of higher density plasmas than those used for MCF, but lower power lasers and other drivers than those used in the ICF approach. The ultimate aim is to significantly drive down costs. MTF has gained a lot of momentum both privately and publicly in recent years, particularly in the US where the US Department of Energy’s ARPA-E ALPHA program has provided support for exploration into the magnetized target fusion route to fusion.
Canada-based General Fusion is one of the most developed MTF players in the space. Building on thirty years of US and Russian Government MTF research, the company has raised over $130 million in funding to develop a 100-200MW unit by 2030. The company has entered industrialization strategy stage, recently partnering with Hatch, an architect/engineering firm, as well as others, to develop a demonstration plant by 2024. This plant will be three quarters the size of a full commercial unit. The team is currently working through a decision process on where to deploy the demo plant, with the largest interest coming out of Canada, the UK and the US.
General Fusion hopes to ultimately produce power at a levelized cost of energy of $50-$60 per MWH, and has drawn up a business model to manufacture a system which can be easily deployed in replacement of fossil-fuel baseload power systems.
Alternative Fuels – A Key Consideration in the Future
The solutions highlighted use deuterium and tritium (DT) as the base fuels. However, taking the DT approach presents some unique problems. While deuterium is a widely abundant material, tritium can only be sourced as a by-product of fission. Ultimately, this means many of the current fusion reactors in development will be dependent on the operation of fission reactors. Furthermore, 80% of the energy in any reactor fueled by DT appears in the form of neutron streams, therefore DT fusion reactors share many of the drawbacks of fission reactors, including the production of large masses of radioactive waste and radiation damage to reactor components.
These problems are endemic to any type of fusion reactor fueled with DT, so abandoning tokamaks for some other confinement concept provides no relief. As a result, other innovators are also looking at alternative fusion fuels, such as the proton-boron-11, or helium-3, which present promising and viable alternatives for long-term use as fuels for fusion energy. One new player in the space is HB11, an Australian-based company which uses hydrogen-boron fuel and a non-thermal approach to fusion. HB11 use lasers as a particle accelerator to accelerate hydrogen or a proton, shooting it through an enriched boron sample to create fusion energy. Currently fundraising for seed investment, they are looking to develop an initial proof of concept, with the promise of being a large fusion contender longer down the line.
Chicken and the Egg
The biggest challenge for both the private and public sector remains that of economically achieving a rate of energy that exceeds the rate of energy injected into the plasma, at scale, over a long period of time. On paper, and with smaller experiments, it seems theoretically possible to sustain net-gain, but this cannot be tested and proven until developers manage to build commercial units. This makes fusion as much of an engineering problem as it a theoretical physical problem. Furthermore, until demonstration plants are built, economical value of fusion systems cannot truly be proven.
Keep an eye on
- It is well worth monitoring other emerging technologies which have not received as much capital. Last year alone, Cleantech Group tracked seven new private fusion energy companies, including German-based Marvel Fusion, Canada-based Type One Energy and France-based Renaissance Fusion to name a few.
- While many see advanced fission as a direct competitor, early commercialization of Generation IV reactors will provide the fusion market with valuable in-field data into how the market will respond to nuclear supplying clean energy to very high temperature applications such as hydrogen production. If you are interested in more details, please check out my previous article here.